Membrane permeability
Simulations at the molecular scale
Molecular modelling is aimed at developing and using simulation techniques to understand biological processes at the molecular scale. The input at the basis of a molecular dynamics (MD) simulation is the description of the interactions between individual atoms. By propagating the system in time or by sampling space with Monte Carlo routines, it becomes possible to see how all those interactions result in larger-scale behavior. The simulations can be extended to larger scales by physics-based theories and this bottom-up approach can thus provide new molecular-level insights in fascinating biological processes. One of the areas for which simulations will be developed and used is membrane research.
The focus of the group is developing new modeling techniques that aid to study molecular behaviors in biomedical research.
Membranes
The studied systems are phospholipid bilayers, which are model systems for biological membranes. A true biological membrane would embed a multitude in different phospholipid types and may be crowded with transmembrane proteins, cholesterol and other molecules, but with the currently available computational resources, membranes are approximated by model system simulations (Fig. 1). Membranes have long been seen as a simple means of compartmentalization of the cell. The transport properties of compounds through and within the membrane are also hypothesized to be related to cell regulation and are now a target for drug development. Membranes have therefore regained an increasing interest in the last two decades.
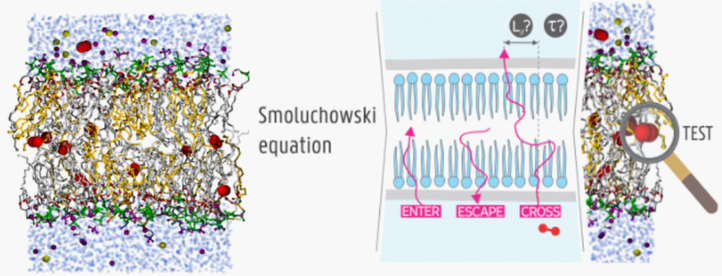
1. Time scale of peptide translocation and flipflop motions
Peptides are short amino acid chains and contain the building blocks of proteins. Peptides are believed to cross the membrane by direct translocation or by forming a micelle-like structure. The governing physics of this process are the interaction between neutral and charged parts of the peptide chain on one hand and the hydrophobic matrix consisting of the phospholipid tails of the membrane on the other hand. Other processes occurring in membranes are a cholesterol flipflop or a lipid flipflop. A lipid or cholesterol molecule embedded in the upper side of the bilayer can flip towards the bottom part of the bilayer and vice versa. This process is very relevant for the (de)mixing of asymmetric bilayers in cells, where the top and bottom layer do not have the same composition in their phospholipid mix. Because these processes are relatively slow compared to available computer power, the time scales for these peptide translocations and flipflop motions are currently not known from standard simulations.
Methods.A new methodology will be developed to reach the time scale of these slow processes. By adding an external bias to molecular dynamics (MD) simulations, the peptide will be pushed through the membrane. Since this destroys the intrinsic dynamics of the peptide translocation, an additional theoretical development is needed to extract the original non-biased dynamics. This will be achieved by assuming an inhomogeneous solubility-diffusive model governed by the Smoluchowski equation which should allow for an estimate of the speed-up caused by the bias potential. The post-processing of the biased MD trajectories should then give the true kinetics of the peptide translocation. The appearance of charges on the peptide will make the computation much more challenging and might require advanced alchemical free energy calculations. For the flipflop motions of cholesterol and lipids, the Smoluchowski treatment will be adapted to describe the rotation of a molecule. The rotation angle will be chosen as a coordinate describing the progression of the flipflop and the theory for characteristic time scales will be converted to be able to describe this non-linear coordinate.
2. Permeability of membranes
Phospholipid bilayers form a barrier for other molecules. Nevertheless, biological function requires compounds to be transported through cells and membranes. An example of passive permeation is the oxygen transport through and inside the membrane, which is the last step in the respiratory cycle before oxygen is consumed by binding to the transmembrane enzyme called cytochrome c oxidase (COX). Previous research on transport through membranes showed the diffusive behavior of oxygen and water in the highly anisotropic and inhomogeneous systems (Fig. 1).
This research on permeability will be extended to more challenging molecules, i.e. small anesthetics and drug molecules, to simulations of active transport through membrane channels, and to transport through liposomes. Opening and blocking of membrane channels is believed to be a route in drug development as it affects the chemical and charge balance in the cell. The kinetics of the permeation through such channels will be investigated.
Methods.
It will be investigated what is the times scale and flux of oxygen given natural oxygen gradients over the membranes. A comparison with the lower oxygen gradients in cancer cells will be made, as local hypoxia is a signature of cancer cells. The oxygen arrival times to reach COX will be estimated based on kinetic rate models in combination with the distribution of these arrival times. It will also be investigated how stacked layers of membranes might function as an oxygen reservoir, as these occur in grey matter in the brain, by simulating both the steady-state and transitional regime.
The experience with oxygen permeability calculations will be extended to permeability simulations of other small molecules, and their paths through the membrane will be analyzed at the molecular level, for instance drug release from liposomes that function as nanocarriers in the cell. A challenging complication is the introduction of a channel in the simulation box. This can be a channel resembling a hollow barrel of stacked proteins, or an artificial ion channel consisting of other partially polar molecules. It will be verified whether the latest polarizable force field models are needed to describe the interactions of the charges and to achieve reliable permeability calculations.
3. Response to membrane distortions
Biological membranes are no solid walls but exhibit a liquid and disordered phase behavior. The question arises how resistant membranes are with respect to structural disruptions, e.g. caused by the passage of drug molecules. Therefore the larger-scale response of membranes to external stimuli will be investigated to assess the mechanical stability of the membranes. The specific envisioned process is the distortion by nanoparticles (NPs), which can be used as carriers for drug delivery by adsorbing drug molecules within the NPs. An innovative application of NPs that is in full development is membrane perforation, where the heating of the particles can increase the membrane's permeability temporarily to allow for better drug transport through the membrane. In some cases, the membrane is completely disrupted by the NP causing cell death, which is a promising feature for cancer research. An experimental setup has been developed at the Faculty of pharmaceutical Sciences at UGent for targeting cancer cells. The process by which such NPs may enhance the permeation is yet to be clarified at the atomic scale, and similarly it is not yet clear how the NPs may completely destroy the membrane.
Methods.
Membrane distortions and defects will be investigated. The mechanical stability of the membrane will be measured by determining the membrane bending modulus from simulations. The bending modulus is based on the moments of the Fourier analysis of the membrane shape and membrane normal vector. The perforation of a membrane by a NP will be modeled explicitly by dragging a NP through the membrane in a steered MD simulation. During this process, the increased leakage of water through the membrane will be measured from permeability calculations. An order parameter will be designed to quantify the membrane defects. It will be investigated how the lipid composition influences the membrane bending modulus, the response to the perforation by the NP, and the associated changes in membrane permeability. This work will also be interpreted in terms of its link with fine dust, where the smaller dust particles can be seen as solid phase NP. Generally, this work will result in a framework for describing large-scale distortions of membranes. This is currently very challenging, because details at the highly inhomogeneous molecular scale are responsible for the distortions that play at a much larger length scale.
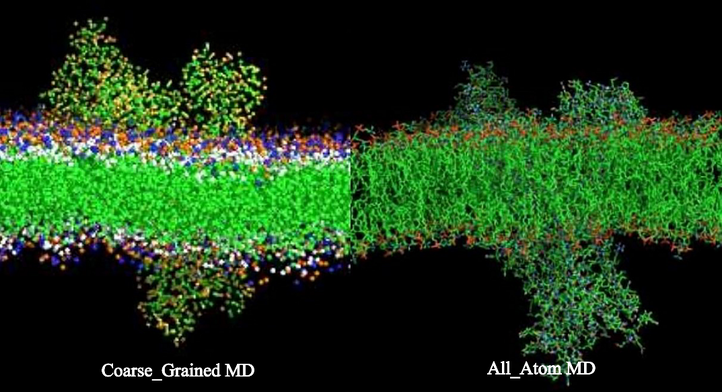
In the all-atom (AA) representation, every atom is represented by a particle. In the coarse-grained (CG) representation, several atoms are lumped together into a larger bead. The CG representation thus lacks the details at the atomic scale, but it has the advantage that the time integration can be performed with a larger time step (10-50 fs) than with the AA representation (1-2 fs).
References
- Enrico Riccardi, Andreas Krämer, Titus S. van Erp and An Ghysels (2020), Permeation Rates of Oxygen through a Lipid Bilayer Using Replica Exchange Transition Interface Sampling. The Journal of Physical Chemistry B. p.193-201. DOI 10.1021/acs.jpcb.0c09947
- Andreas Krämer, An Ghysels, Eric Wang, Richard M. Venable, Jeffery B. Klauda, Bernard R. Brooks and Richard W. Pastor (2020), Membrane permeability of small molecules from unbiased molecular dynamics simulations. The Journal of Chemical Physics. DOI 10.1063/5.0013429
- Samaneh Davoudi and An Ghysels (2021), Sampling efficiency of the counting method for permeability calculations estimated with the inhomogeneous solubility–diffusion model. The Journal of Chemical Physics. DOI 10.1063/5.0033476
- An Ghysels, Andreas Krämer, Richard M. Venable, Walter E. Teague, Edward Lyman, Klaus Gawrisch and Richard W. Pastor (2019), Permeability of membranes in the liquid ordered and liquid disordered phases. Nature communications. 10. DOI 10.1038/s41467-019-13432-7
- Oriana De Vos, Tanja Van Hecke and An Ghysels (2018), Effect of chain unsaturation and temperature on oxygen diffusion through lipid membranes from simulations. Advances in experimental medicine and biology. 1072. p.399-404. DOI 10.1007/978-3-319-91287-5_64
- Oriana De Vos, Richard Venable, Tanja Van Hecke, Gerhard Hummer, Richard Pastor and An Ghysels (2018), Membrane permeability: characteristic times and lengths for oxygen, and a simulation-based test of the inhomogeneous solubility-diffusion model. Journal of chemical theory and computation. 14(7). p.3811-3824. DOI 10.1021/acs.jctc.8b00115
- An Ghysels, Richard M Venable, Richard W Pastor and Gerhard Hummer (2017), Position-dependent diffusion tensors in anisotropic media from simulation: oxygen transport in and through membranes.Journal of chemical theory and computation. 13(6). p.2962-2976. DOI 10.1021/acs.ictc.7b00039